This study develops and evaluates a novel polymeric nanoparticle system for the delivery of ruthenium-based cytotoxic agents, demonstrating enhanced tumor targeting, reduced off-target toxicity, and promising efficacy against high-grade ovarian cancer, including in cisplatin-resistant models.
Key Preview
Research Question
- The study investigates the challenges of effectively delivering ruthenium-based cytotoxic agents, focusing on overcoming pharmacokinetic limitations such as stability, off-target toxicity, and poor membrane permeability to enhance the therapeutic efficacy of ruthenium drugs in treating high-grade epithelial ovarian cancer.
Research Design and Strategy
- The research employs a one-pot synthesis process for ruthenium-polylactide nanoparticles using ring-opening polymerization initiated by a calcium alkoxide derivative, aiming to create a stable and customizable drug delivery system that enhances drug accumulation in tumors while minimizing toxicity to healthy tissues.
Method
- Ruthenium complexes are synthesized with specific ligands and covalently attached to polylactide through controlled polymerization; the resulting nanoparticles are characterized for size, drug loading, and release kinetics, and tested in vitro on ovarian cancer cell lines and in vivo on murine models for pharmacokinetics and biodistribution.
Key Results
- The nanoparticles demonstrated a controlled release of the ruthenium payload with up to 68% drug loading, showing cytotoxicity similar to cisplatin, enhanced cellular uptake (over 200 times greater than free ruthenium), and improved tumor accumulation by 18-fold while reducing off-target toxicity by up to 97%.
Significance of the Research
- This study offers a promising approach to overcoming drug delivery challenges, potentially advancing the use of ruthenium complexes for effective cancer treatments, particularly for high-grade ovarian cancer, by leveraging the benefits of polymeric nanoparticle systems in enhancing drug stability, pharmacokinetics, and targeted delivery.
Introduction
High-grade epithelial ovarian cancer remains one of the most lethal gynecological cancers, with a high mortality rate and limited effective treatment options, particularly for patients exhibiting platinum resistance. The evolution of cancer therapeutics has seen an increased interest in metal-based drugs, particularly those involving ruthenium, due to their promising cytotoxic properties. However, despite the potential benefits, the clinical application of these compounds has been hampered by significant pharmacokinetic challenges, including low solubility and rapid clearance from the bloodstream. This research addresses these critical issues by investigating a novel polymeric nanoparticle system designed for the safe and controlled delivery of ruthenium complexes. The study aims to enhance drug stability, increase cellular uptake, and improve therapeutic outcomes, ultimately leading to more effective treatment strategies for advanced ovarian cancer.
Research Team and Objective
The research team, composed of João P. M. António, Albert Gandioso, Fariba Nemati, Nancy Soliman, Robin Vinck, Fan Sun, Carine Robert, Pierre Burckel, Didier Decaudin, Christophe M. Thomas, and Gilles Gasser, conducted this study at Chimie ParisTech, PSL University, and the Institut Curie. The paper, titled “Polymeric encapsulation of a ruthenium(II) polypyridyl complex: from synthesis to in vivo studies against high-grade epithelial ovarian cancer,” was published in the journal Chemical Science. The objective of this research was to develop a biocompatible and effective delivery system that could enhance the therapeutic potential of ruthenium-based drugs, addressing the urgent need for better treatment options in ovarian cancer.
Experimental Process
Outline:
1. Synthesis of Ruthenium Complexes
2. Polymerization and Synthesis of Ruthenium-Polylactide Complex
3. Formation and Characterization of Nanoparticles
4. In Vitro Cytotoxicity Testing
5. In Vivo Pharmacokinetics and Biodistribution
1. Synthesis of Ruthenium Complexes
Key Steps:
The first step of the process involves synthesizing a ruthenium(II) complex with the desired ligand architecture. Specifically, a ruthenium complex featuring a hydroxymethyl-functionalized phenyl-1H-imidazo-1,10-phenanthroline ligand was designed. The synthesis began with the preparation of the ruthenium complex Ru(2,2′-bipyridine)2(4-hydroxymethyl-phenyl-1H-imidazo-1,10-phenanthroline)2 (referred to as “Ru”). This compound was synthesized by a multi-step organic reaction pathway, including the formation of a bipyridine-ruthenium complex and subsequent attachment of the hydroxymethyl group on the phenyl ring.
Results and Key Data:
The successful synthesis was confirmed by several analytical techniques, including NMR spectroscopy, UV-vis spectrophotometry, and high-resolution mass spectrometry. The complex showed stable characteristics, with the ruthenium center remaining intact in both organic and aqueous phases. Notably, the ruthenium complex was stable in plasma for over 10 days, demonstrating promising stability for use in biological applications.
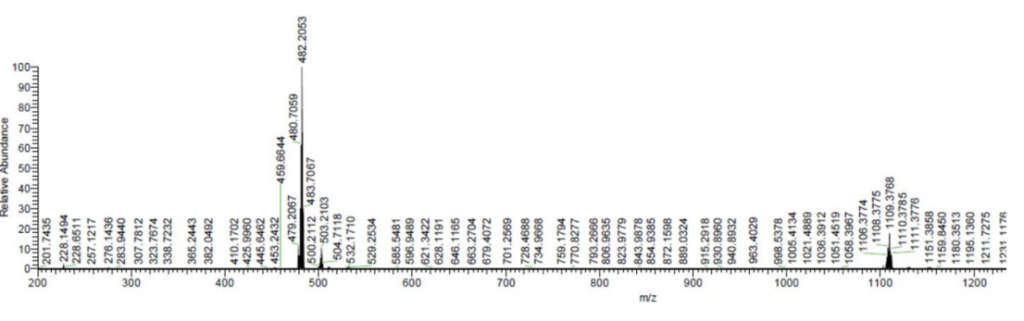
Figure 1. HRMS spectrum of Ru.
Significance of the Results:
The ability to synthesize a stable ruthenium complex with a hydroxymethyl group as a functional handle is critical for the subsequent attachment of the complex to the polylactide polymer. This step addresses the challenge of ensuring both the chemical stability of the ruthenium complex and its compatibility with the drug delivery system, which is essential for effective therapeutic applications.
Key Innovations:
This approach highlights the novel use of a hydroxymethyl group as a functional handle for initiating polymerization, overcoming the limitations posed by less stable functional groups in previous methods. This modification improves the overall stability and bioavailability of the ruthenium complex.
2. Polymerization and Synthesis of Ruthenium-Polylactide Complex
Key Steps:
The next step involves the polymerization of lactide (LA) using a calcium-based catalyst to form polylactide (PLA), a biodegradable polymer. A key modification in this procedure is the use of a biocompatible calcium alkoxide catalyst (Ca[N(SiMe3)2]2·2THF) in place of traditional toxic catalysts like tin(II) bis(2-ethylhexanoate) (Sn(Oct)2). The ruthenium complex (Ru) is incorporated into the polymer backbone via ring-opening polymerization (ROP) of lactide. This step is performed in a 1:1 solvent system of tetrahydrofuran (THF) and dichloromethane (CH2Cl2) to maintain the solubility of the catalyst and the ruthenium complex, initiating polymerization at room temperature.
Results and Key Data:
The polymerization proceeded with near-quantitative conversion (>96%) and successful incorporation of the ruthenium complex into the polymer chain. Four different polymers (P1–P4) were synthesized, each with varying molecular weights ranging from 2 kDa to 11 kDa. The ruthenium loading in the polymers up to 68% by weight, confirming efficient incorporation of the cytotoxic agent.
Significance of the Results:
This step is crucial in ensuring the successful integration of the ruthenium complex into a stable polymeric carrier that can release the drug in a controlled manner. By achieving high incorporation efficiency and a range of molecular weights, the study addresses the need for a customizable drug delivery system capable of optimizing therapeutic efficacy while minimizing toxicity.
Key Innovations:
The use of a non-toxic calcium catalyst and a simple, one-pot polymerization process represents a significant advancement over traditional methods, which often involve complex purification steps and the use of hazardous materials. This innovation simplifies the synthesis process and makes it more suitable for biomedical applications.
3. Formation and Characterization of Nanoparticles
Key Steps:
Once the ruthenium-polylactide polymers were synthesized, they were formulated into nanoparticles through a modified nanoprecipitation method. The polymer was dissolved in acetone and mixed dropwise with an aqueous solution of Kolliphor P188, a surfactant that stabilizes the nanoparticles. The solvent was evaporated under reduced pressure to form a nanoparticle suspension, which was further purified by centrifugation to remove large aggregates.
Results and Key Data:
Dynamic light scattering (DLS) measurements revealed that the nanoparticles (NP1–NP4) had an average size range between 104 and 134 nm, ideal for passive targeting to tumors via the enhanced permeability and retention (EPR) effect.

Table 1.Properties of NP1–NP4
Transmission electron microscopy (TEM) confirmed the nanoparticles had a spherical shape with diameters of approximately 110 nm.
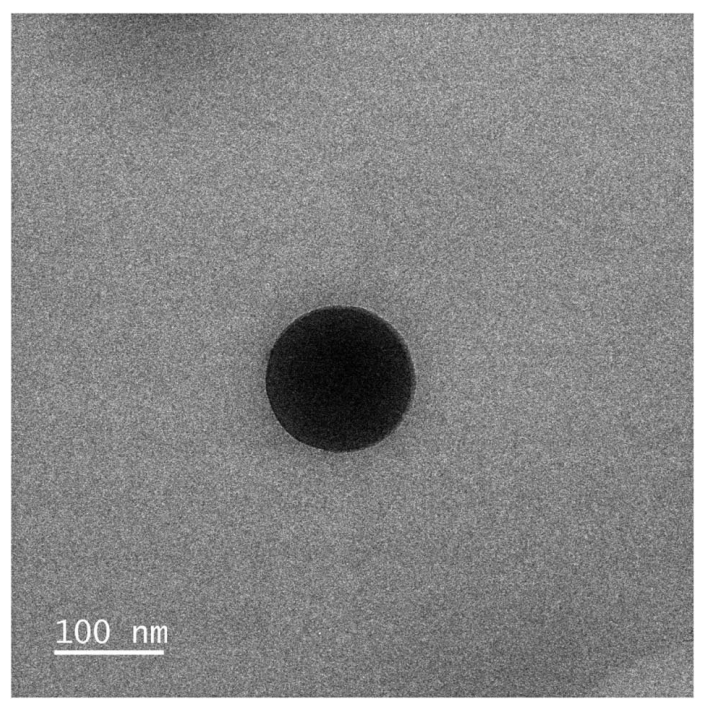
Figure 2. TEM image of NP3 showing a spherical nanoparticle with a diameter of approximately 100 nm.
The zeta potential of NP3 was measured at +80 mV, indicating good electrostatic stability of the nanoparticles.
Significance of the Results:
The successful formation of stable nanoparticles with controlled size and high stability in aqueous environments is essential for ensuring that the drug delivery system remains effective in biological systems. The particle size is small enough to facilitate tumor penetration but large enough to avoid rapid elimination from the bloodstream.
Key Innovations:
The ability to control the size distribution and achieve high stability with simple processing methods is a significant innovation. The use of a non-toxic surfactant (Kolliphor P188) in combination with the one-pot polymerization technique streamlines the synthesis process and provides a more efficient and biocompatible platform for drug delivery.
4. In Vitro Cytotoxicity Testing
Key Steps:
The next phase involved evaluating the cytotoxicity of the synthesized nanoparticles against ovarian cancer cell lines (A2780, A2780 cisplatin-resistant) using a fluorometric resazurin assay. The nanoparticles were incubated with the cells for 48 hours at concentrations ranging from 1 to 100 µM. The cell viability was measured, and IC50 values were calculated for the nanoparticles and the free ruthenium complex.
Results and Key Data:
The nanoparticles showed cytotoxicity in the low micromolar range, with IC50 values of 24.8 µM (NP1) and 35.4 µM (NP3) after 48 hours. Notably, the cytotoxicity was maintained even in the cisplatin-resistant A2780 cell line, indicating a potentially orthogonal mechanism of action. In contrast, the free ruthenium complex exhibited higher toxicity in the A2780 cell line, with an IC50 of 6.6 µM.
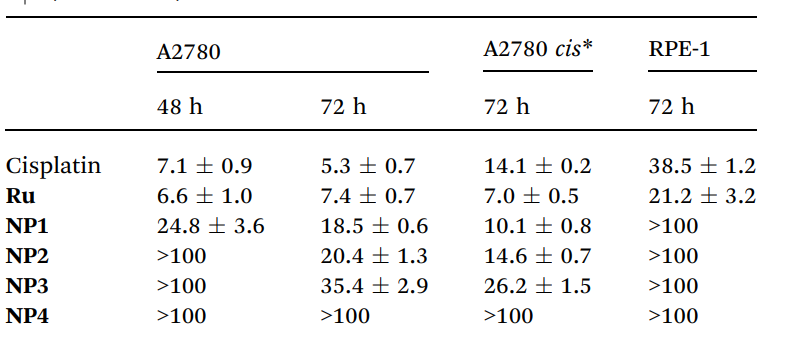
Table 2.IC50 [mM] values for Ru, NP1–NP4, and cisplatin in three different cell lines (48 and 72 h). Representative data from three independent experiments are shown
Significance of the Results:
The ability of the nanoparticles to maintain or even enhance cytotoxicity in a cisplatin-resistant cell line is highly significant, as it suggests that the ruthenium-loaded nanoparticles could offer a promising alternative for treating platinum-resistant ovarian cancer, which is a critical clinical challenge.
Key Innovations:
The study demonstrated the use of polymeric nanoparticles to enhance the drug’s cellular uptake, achieving significantly higher drug concentrations inside the cells compared to free ruthenium. This result highlights the importance of nanoparticle-mediated drug delivery in overcoming drug resistance.
5. In Vivo Pharmacokinetics and Biodistribution
Key Steps:
For in vivo evaluation, murine models were used to assess the pharmacokinetics and biodistribution of the nanoparticles. Mice were injected intravenously with NP3, and ruthenium content in various organs was quantified using inductively-coupled plasma mass spectrometry (ICP-MS). Tumor accumulation was assessed, and off-target effects in organs such as the liver, kidneys, and lungs were measured.
Results and Key Data:
The nanoparticles demonstrated an 18-fold increase in drug accumulation in tumor tissues compared to free ruthenium. Furthermore, the accumulation of ruthenium in peripheral organs such as the brain and lungs was reduced by up to 97%, significantly lowering the risk of systemic toxicity. The nanoparticles were well tolerated by the mice, with no significant weight loss or other adverse effects observed.
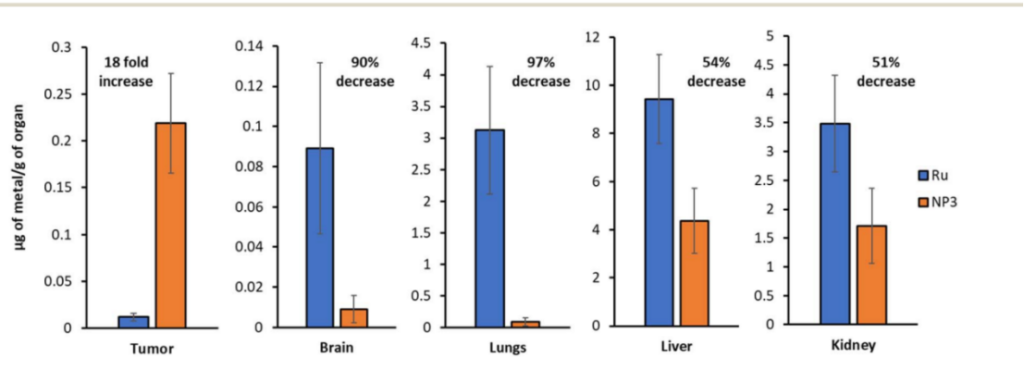
Figure 3.ICP-MS quantification of the ruthenium content in various organs (Ru vs. NP3). Representative data from five independent experiments is shown.
Significance of the Results:
The improved tumor accumulation and reduced off-target toxicity confirm the potential of ruthenium-loaded nanoparticles to deliver higher concentrations of the drug to cancerous tissues while minimizing side effects. This outcome validates the nanoparticle platform as an effective drug delivery system.
Key Innovations:
This study introduces a novel nanoparticle formulation with significantly improved pharmacokinetics, highlighting the importance of covalent encapsulation in enhancing drug stability and controlled release in vivo. The in vivo findings provide strong evidence for the future clinical potential of the ruthenium-polylactide nanoparticle system.
Conclusion
The study concluded that the polymeric encapsulation of ruthenium(II) complexes represents a promising strategy for enhancing the treatment of high-grade epithelial ovarian cancer. Key findings indicated that the developed nanoparticles not only improved drug stability and release control but also significantly increased drug accumulation in tumors while minimizing systemic toxicity. However, the research also acknowledged limitations regarding the inherent cytotoxicity of the ruthenium complex itself, suggesting that future efforts should focus on optimizing the cytotoxic payload. Continued exploration into the application of this nanoparticle technology could lead to significant advancements in cancer therapeutics, potentially extending its use to various other malignancies in need of effective treatment options.
Reference:
António, João PM, et al. “Polymeric encapsulation of a ruthenium (ii) polypyridyl complex: from synthesis to in vivo studies against high-grade epithelial ovarian cancer.” Chemical Science 14.2 (2023): 362-371.