Editor: Nina
This study investigates how different formulations of self-amplifying RNA (saRNA) vaccines influence immune responses by modulating inflammation and antigen expression, revealing that lipid nanoparticle formulations elicit the strongest adaptive immunity, offering insights for optimizing RNA vaccine design.
Key Preview
Research Question
- How do different formulations of self-amplifying RNA (saRNA) vaccines influence the balance between inflammation and antigen expression, thereby affecting immune responses?
Research Design and Strategy
- A comparative analysis of saRNA vaccine formulations, including cationic polymers, lipid emulsions, and lipid nanoparticles (LNPs), to investigate their effects on systemic cytokine responses and adaptive immunity.
Method
- Intramuscular immunization of C57BL/6 mice with varying saRNA formulations followed by measurement of cytokine levels and antibody responses.
Key Results
- LNP-formulated saRNA vaccines induced significantly higher levels of cytokines and antibody responses compared to other formulations. The correlation between cytokine response and antibody production highlights the role of inflammation in immune activation.
Significance of the Research
- The findings advance the understanding of how saRNA vaccine formulations affect immune responses, offering insights for optimizing RNA vaccine designs by balancing beneficial inflammation with effective antigen expression.
Introduction
The advent of the COVID-19 pandemic has catalyzed remarkable advancements in vaccine technology, with RNA-based vaccines emerging as a flexible and rapid response platform for infectious diseases. The initial deployment of these vaccines illustrated their potential, yet challenges remain in achieving consistent immune responses across diverse populations. Self-amplifying RNA (saRNA) vaccines represent an innovative approach, leveraging the replicative nature of alphaviruses to enhance antigen expression and potentially reduce the required dose for effective immunity. However, the variability in immune responses observed in clinical trials underscores the necessity of understanding the underlying mechanisms that govern efficacy.
This research investigates the interplay between saRNA vaccine formulation, inflammation, and the resulting adaptive immune response. Specifically, it seeks to address questions regarding how different formulations impact the systemic cytokine response and how this correlates with antibody production. Such understanding is vital for optimizing vaccine design, particularly as RNA technologies continue to advance in both therapeutic and prophylactic applications.
Research Team and Objective
The research team is comprised of experts from Imperial College London and various institutions, including John S. Tregoning, David C. Stirling, Ziyin Wang, Katie E. Flight, Jonathan C. Brown, Anna K. Blakney, Paul F. McKay, Robert F. Cunliffe, Valarmathy Murugaiah, Christopher B. Fox, Mitchell Beattie, Ying K. Tam, Cecilia Johansson, and Robin J. Shattock. Conducted between early 2022 and late 2022, this study was published in the journal Molecular Therapy: Nucleic Acids. The primary objective of the research is to investigate how different formulations of saRNA vaccines influence immune responses, particularly focusing on the balance between the inflammatory response and the expression of vaccine antigens, thus contributing to the field of virology and vaccine development.
Experimental Process
Outline:
- Vaccine Formulation Preparation
- Mouse Immunization and Cytokine Measurement
- Antibody Response Assessment
- Investigating Innate Immune Sensing
- Data Integration and Analysis
1. Vaccine Formulation Preparation
Key Steps:
- Researchers prepared three different formulations of self-amplifying RNA (saRNA) vaccines: a cationic polymer (pABOL), a lipid emulsion (nanostructured lipid carrier, NLC), and three lipid nanoparticles (LNPs).
- The pABOL formulation was created using a titration method to generate particles of ~200 nm with a slight positive charge (5 mV zeta potential).
- NLC formulations were synthesized through a combination of heated oil and aqueous phases, followed by high-pressure homogenization, resulting in ~40 nm particles with a strongly positive charge (28 mV).
- LNPs were rapidly mixed using an ethanolic lipid solution and saRNA in an acidic buffer, yielding particles ~85 nm in size with neutral surface charge and high encapsulation efficiency (>90%).
Results and Key Data:
- Particle size and charge measurements confirmed that the formulations were stable and appropriate for cellular uptake and saRNA delivery.
- Encapsulation efficiencies were consistently above 90%, ensuring optimal RNA delivery.
Significance of the Results:
- These formulations represent diverse delivery systems that facilitate RNA uptake and provide a comparative platform to study their immunogenic effects.
- The particle characteristics are critical for determining the balance between inflammation and antigen expression, a central research question.
Key Innovations:
- The preparation of highly stable, well-characterized saRNA formulations with distinct physical properties allowed for precise comparisons of immune responses.
- Incorporating three LNP formulations with varying lipid compositions expanded insights into optimal vaccine delivery strategies.
2. Mouse Immunization and Cytokine Measurement
Key Steps:
- Female C57BL/6 mice were immunized intramuscularly with saRNA vaccines at different doses (0.1 µg, 1 µg, 10 µg).
- Blood samples were collected 4 hours post-immunization to assess acute cytokine responses using a multiplex cytokine assay.
- Cytokines measured included IL-6, IP-10, MCP-1, and others indicative of systemic inflammation.
Results and Key Data:
- LNP formulations induced significantly higher cytokine levels compared to pABOL and NLC formulations. For example, 10 µg LNP formulations led to elevated IL-6 (~10-fold increase over pABOL) and IP-10 (~8-fold increase).
- A dose-response relationship was observed, with higher doses correlating with stronger cytokine responses.
Significance of the Results:
- These findings demonstrated that cytokine levels are predictive of the adaptive immune response.
- The results highlight the dual role of inflammation in boosting immune activation and influencing vaccine effectiveness.
Key Innovations:
- The systematic evaluation of cytokine profiles provided direct evidence of the link between acute inflammation and adaptive immunity.
- The use of multiplex cytokine assays enabled high-throughput, quantitative analysis of inflammatory markers.
3. Antibody Response Assessment
Key Steps:
- Serum samples were collected at 4 and 6 weeks post-immunization to measure antigen-specific IgG antibody levels using ELISA.
- Mice were also challenged with H1N1 influenza virus to evaluate protection.
Results and Key Data:
- LNP formulations elicited the highest antibody titers, with a 2–3-fold increase compared to NLC and pABOL.
- Mice immunized with 10 µg LNP-formulated saRNA exhibited complete protection against weight loss and viral load after H1N1 infection.
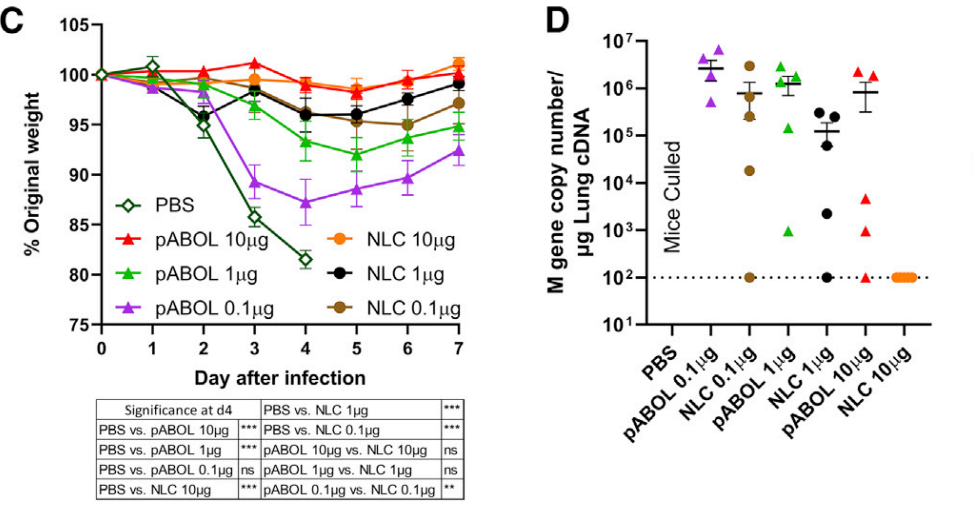
Figure 1. Mice were infected intranasally with influenza virus at 6 weeks, and weight loss was measured after infection (C) and viral load at day 7 after infection (D).
- Lower doses of LNP (e.g., 0.1 µg) provided protection equivalent to higher doses of pABOL, highlighting their potency.
Significance of the Results:
- The antibody response correlated strongly with cytokine levels, confirming the role of inflammation in enhancing vaccine-induced immunity.
- These results directly address the research objective of identifying optimal saRNA formulations for robust immune responses.
Key Innovations:
- The use of dose-sparing strategies demonstrated that LNP formulations can achieve high efficacy with minimal RNA quantities, a critical advancement for scalability in pandemic scenarios.
- Combining immunogenicity data with viral challenge experiments provided comprehensive validation of vaccine effectiveness.
4.Investigating Innate Immune Sensing
Key Steps:
- Mice with genetic knockouts for key innate immune signaling pathways (e.g., MAVS, MyD88/TRIF, IFNAR1) were immunized with LNP-formulated saRNA.
- Cytokine responses and adaptive immune outcomes were compared to wild-type mice.
Results and Key Data:
- MAVS-deficient mice displayed significantly higher antibody titers (~30% increase) and reduced cytokine levels (e.g., ~50% decrease in IL-6).
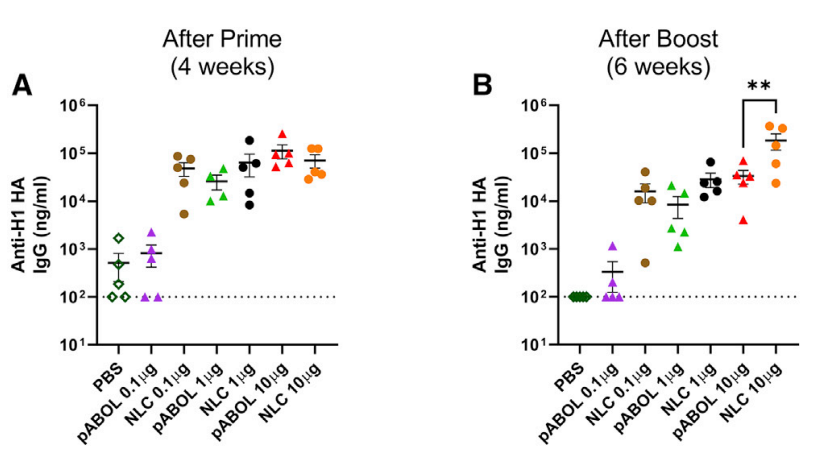
Figure 2. Blood was collected to measure anti-HA antibody responses at 4 (A) and 6 weeks (B)
- MyD88/TRIF-deficient mice exhibited attenuated cytokine responses, while IFNAR1-deficient mice showed enhanced T-cell responses.
Significance of the Results:
- These experiments revealed that certain inflammatory pathways (e.g., MAVS and MyD88/TRIF) can inhibit or enhance adaptive immunity.
- The results underscore the importance of fine-tuning innate immune signaling to optimize RNA vaccine design.
Key Innovations:
- The use of knockout mouse models provided mechanistic insights into the relationship between innate sensing and adaptive immunity.
- These findings highlight potential targets for modulating inflammation to improve vaccine efficacy.
5. Data Integration and Analysis
Key Steps:
- Cytokine and antibody response data were pooled across experiments to establish correlations.
- Statistical analyses were conducted to identify significant predictors of vaccine effectiveness.
Results and Key Data:
- Cytokine markers such as IP-10, MCP-1, and IL-6 showed strong correlations with antibody titers.
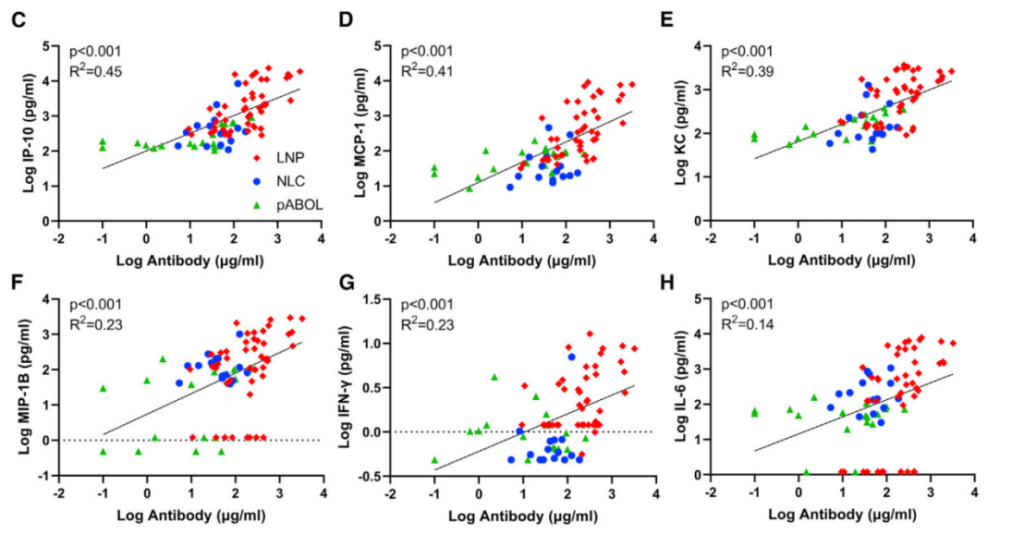
Figure 3. Correlations for IP-10 (C), KC (D), MCP-1 (E), MIP-1B (F), IFN-g (G), and IL-6 (H).
- LNPs consistently outperformed other formulations in all key metrics of immunogenicity and protection.
Significance of the Results:
- The integrated data confirmed the hypothesis that inflammation drives adaptive immune responses in saRNA vaccination.
- These results provide a roadmap for designing saRNA vaccines that balance inflammation with antigen expression.
Key Innovations:
- The comprehensive approach combined quantitative and qualitative analyses to uncover complex immune interactions.
- The study’s framework can be adapted to evaluate other RNA vaccine platforms, expanding its impact on the field.
Conclusion
In conclusion, the research findings underscore the intricate relationship between saRNA vaccine formulation, inflammation, and adaptive immune responses. The study reveals that formulations provoking a robust cytokine response are associated with enhanced antibody production and protection against viral challenges. Future research should focus on exploring the specific pathways through which different formulations influence immune responses and investigating the implications for human vaccine development. This will be critical for optimizing RNA vaccine strategies to ensure effective and long-lasting immunity in diverse populations, particularly in the face of emerging infectious diseases.
Reference:
Tregoning, John S., et al. “Formulation, inflammation, and RNA sensing impact the immunogenicity of self-amplifying RNA vaccines.” Molecular Therapy-Nucleic Acids 31 (2023): 29-42.