Researchers from King Abdulaziz University have shown that Parthenolide phytosomes can significantly alleviate kidney damage caused by gentamicin by addressing oxidative stress, inflammation, and apoptosis.
Key Highlights:
Research Question
- Can Parthenolide phytosomes effectively mitigate gentamicin-induced nephrotoxicity by enhancing bioavailability and targeting oxidative stress, inflammation, and apoptosis in the kidneys?
Research Difficulties
- Developing a stable and effective phytosome formulation to enhance Parthenolide’s poor bioavailability and solubility.
- Addressing the complex and multifactorial nature of gentamicin-induced nephrotoxicity, which involves oxidative stress, inflammation, and apoptosis.
Key Findings
- Parthenolide phytosomes significantly reduced serum levels of kidney injury markers (urea by 25.1–29.5%, creatinine by 17.5–35.1%, and cystatin C by 37.1–50.1%).
- Restored antioxidant enzyme activity (SOD by 33.3–47.6%, CAT by 45.1–58.8%) and reduced lipid peroxidation (MDA by 34.6–46.2%).
- Histological analysis revealed reduced tubular necrosis, inflammation, and fibrosis, with the kidney injury score decreasing from 4.2 (gentamicin-treated) to 0.5 (high-dose phytosome treatment).
- Activated key molecular pathways, including Sirt-1, Nrf2, HO-1, and NQO1, to counteract oxidative stress and apoptosis.
Innovative Aspects
- First use of Parthenolide phytosomes as a nanotechnology-based therapeutic approach for nephrotoxicity.
- Demonstrated enhanced bioavailability and stability of Parthenolide through phytosome formulation.
Importance of the Study
- This study addresses the critical need for effective nephroprotective therapies in patients undergoing gentamicin treatment. The findings not only highlight the potential of Parthenolide phytosomes as a novel therapeutic strategy but also pave the way for using phytosome-based formulations to treat other oxidative stress-related renal injuries.
Challenges in Addressing Gentamicin-Induced Kidney Injury
The Clinical Burden of Gentamicin Nephrotoxicity
Gentamicin, a widely prescribed antibiotic for bacterial infections, carries the significant drawback of nephrotoxicity, which occurs in nearly 30% of patients receiving prolonged treatment. The condition often manifests through elevated serum creatinine and urea levels, tubular necrosis, and severe oxidative stress, leading to impaired kidney function and increased healthcare challenges.
Therapeutic Limitations in Nephrotoxicity
Existing treatment strategies for nephrotoxicity primarily focus on managing symptoms through antioxidants and anti-inflammatory agents. These approaches, while somewhat effective, often fail to comprehensively mitigate the underlying oxidative and inflammatory mechanisms, leaving room for improved therapeutic options.
Potential of Parthenolide
Parthenolide, a compound extracted from the feverfew plant (Tanacetum parthenium), has been noted for its antioxidant and anti-inflammatory effects. However, its clinical application has been hindered by poor solubility and limited bioavailability, highlighting the need for advanced delivery methods to unlock its therapeutic potential.
Objectives and Approach of the Study
Research Focus
The study aimed to determine whether Parthenolide phytosomes, a novel lipid-based nanotechnology, could address gentamicin-induced nephrotoxicity by enhancing Parthenolide’s bioavailability and effectively targeting kidney damage at molecular and cellular levels.
Phytosomal Design
Using solvent evaporation techniques, researchers encapsulated Parthenolide into phytosomes, creating nanoparticles with an average size of 407.4 nm. This design improved solubility and ensured better absorption, allowing for enhanced therapeutic efficacy.
Study Context
Conducted by a team from King Abdulaziz University, the findings were published in the journal Molecules on March 17, 2023. This study stands as a preclinical assessment, offering a foundation for future exploration of phytosome-based formulations.
Experimental Work and Observations
Experimental Process Overview
- Preparation of Parthenolide phytosomes using solvent evaporation and nanoparticle formulation.
- Division of rats into six treatment groups, including controls, gentamicin-treated, and phytosome-treated groups at two dosage levels (5 mg/kg and 10 mg/kg).
- Evaluation of kidney function markers, oxidative stress levels, histopathological changes, and molecular pathway activation.
Detailed Key Experiments and Findings
- Phytosome Preparation and Characterization
- Preparatory Work: Parthenolide (99.2 mg) was combined with phosphatidylcholine (376.8 mg) to create a phytosomal formulation. The compounds were dissolved in ethanol and chloroform, stirred thoroughly, and evaporated to form a thin lipid film. The film was hydrated with distilled water and subjected to ultrasonication to ensure uniform nanoparticle formation.
- Steps: The prepared phytosomes were analyzed for particle size, polydispersity index (PDI), and morphology using dynamic light scattering and Transmission Electron Microscopy (TEM).
- Results: The phytosomes exhibited a z-average particle size of 407.4 nm, a PDI of 0.6 (indicating homogeneity), and an oval, multi-edged surface morphology as confirmed by TEM imaging.
- Effects on Kidney Function Markers
- Preparatory Work: Gentamicin (100 mg/kg) was administered intraperitoneally to rats for seven days to induce nephrotoxicity. Blood samples were collected from all experimental groups, including controls and Parthenolide phytosome-treated groups.
- Steps: Serum urea, creatinine, and cystatin C levels were analyzed to assess kidney function across treatment groups.
- Results: Gentamicin significantly elevated serum urea by 133.3%, creatinine by 217.9%, and cystatin C by 200% compared to the control. Treatment with Parthenolide phytosomes reduced these markers by 25.1–50.1%, demonstrating a significant improvement in kidney function.
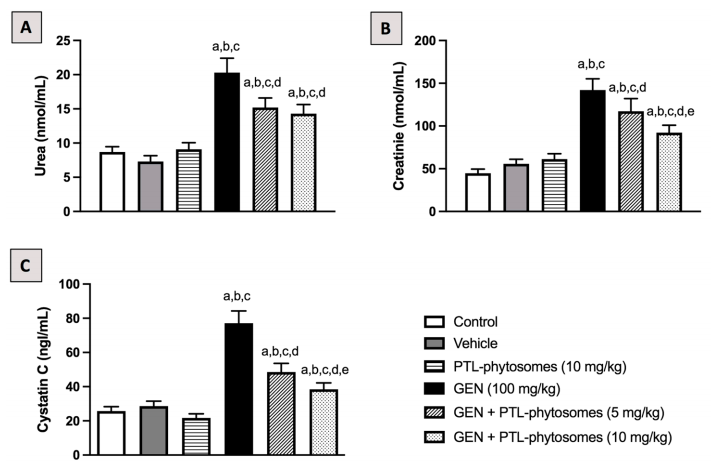
- Impact on Oxidative Stress
- Preparatory Work: Rat kidney tissues were harvested after treatment, and homogenized tissue samples were prepared to measure markers of oxidative stress, including malondialdehyde (MDA), superoxide dismutase (SOD), and catalase (CAT).
- Steps: MDA levels were measured to evaluate lipid peroxidation, while SOD and CAT activities were assessed to gauge antioxidant enzyme activity.
- Results: Gentamicin increased MDA levels by 136.3% and decreased SOD and CAT activities by 34.4% and 38.5%, respectively, compared to controls. Parthenolide phytosome treatment reversed these effects, reducing MDA by 34.6–46.2% and increasing SOD and CAT activities by 33.3–58.8%.
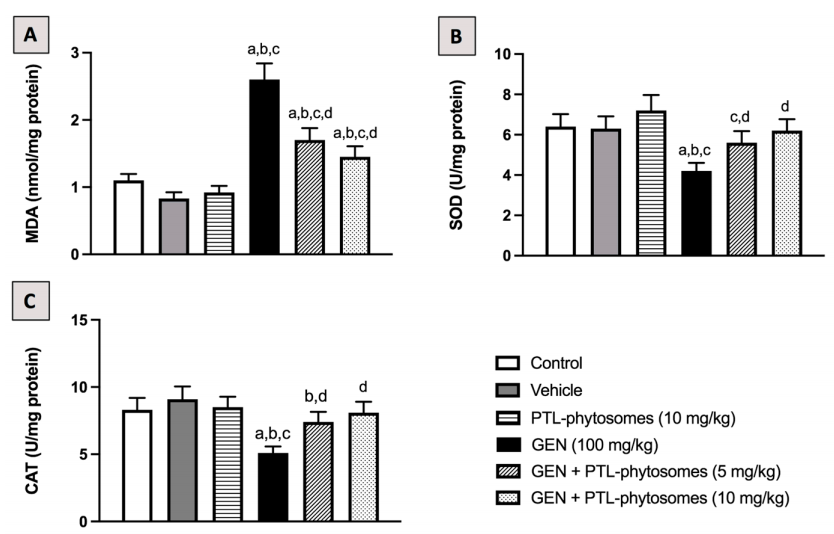
- Histopathological Improvements
- Preparatory Work: Kidney tissues were collected and fixed for histological analysis. Staining methods such as hematoxylin and eosin (H&E), periodic acid-Schiff (PAS), and Masson’s Trichrome were used to evaluate tubular necrosis, inflammation, and fibrosis.
- Steps: Tissue sections were examined microscopically to quantify kidney injury and assess the structural damage in tubular and glomerular regions.
- Results: Gentamicin-treated kidneys exhibited severe tubular necrosis, inflammatory infiltration, and interstitial fibrosis, with a kidney injury score of 4.2. Parthenolide phytosome treatment significantly reduced these pathological changes, with the score decreasing to 0.5 in the high-dose treatment group.
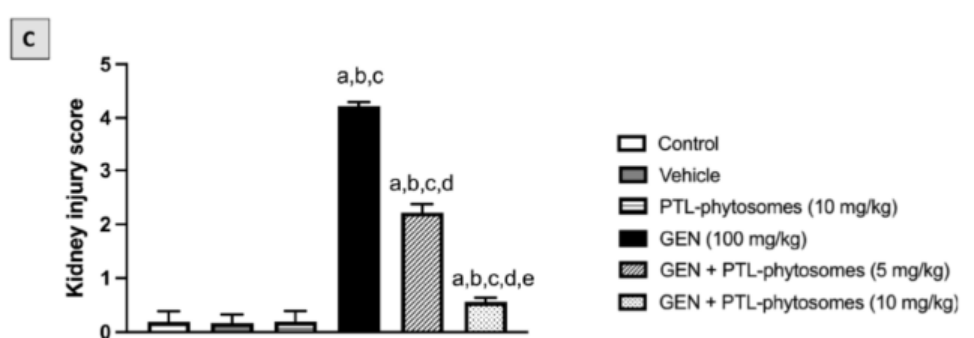
- Molecular Pathway Activation
- Preparatory Work: Protein extracts from kidney tissue were prepared for Western blot analysis to evaluate the expression levels of Sirt-1, Nrf2, HO-1, and NQO1, which are key markers of antioxidant defense pathways.
- Steps: Western blot analysis was conducted to determine changes in protein expression levels in response to gentamicin and Parthenolide phytosome treatment.
- Results: Gentamicin significantly reduced the expression of Sirt-1, Nrf2, HO-1, and NQO1 by 73.4–91.9% compared to the control group. Treatment with Parthenolide phytosomes restored these levels, increasing protein expression by 207.9–612%, indicating activation of antioxidant and cytoprotective pathways.
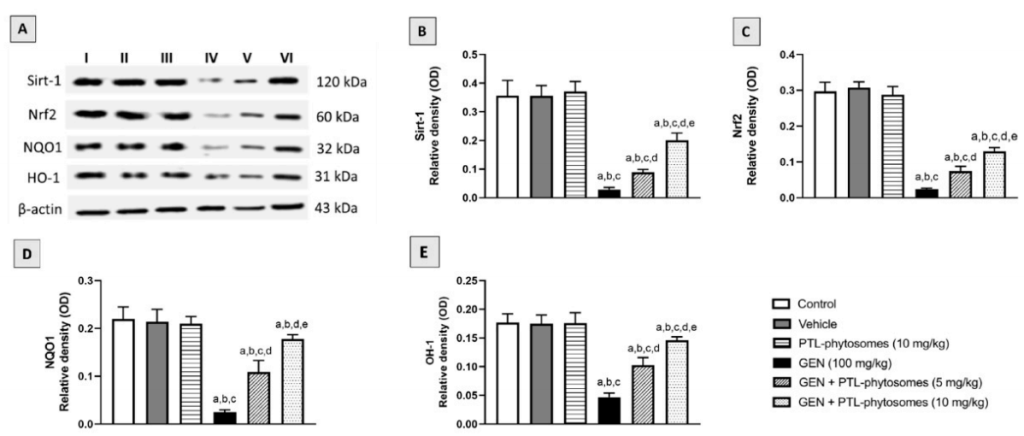
The results clearly show that Parthenolide phytosomes effectively mitigate gentamicin-induced nephrotoxicity through multiple mechanisms. The phytosomal formulation improved kidney function by lowering biomarkers such as serum urea, creatinine, and cystatin C, and restored antioxidant enzyme activity. It also reduced oxidative damage, as evidenced by lower MDA levels and higher SOD and CAT activities. Histological analysis confirmed reduced tissue damage, including inflammation, necrosis, and fibrosis, while molecular analysis demonstrated the activation of key protective pathways, such as Sirt-1, Nrf2, HO-1, and NQO1. Together, these findings establish Parthenolide phytosomes as a promising therapeutic intervention for kidney injury.
Significance and Broader Implications
The use of phytosome nanotechnology to enhance Parthenolide’s bioavailability represents a notable step forward in addressing drug delivery challenges for nephroprotective agents.
This study addresses the unmet need for effective nephroprotective therapies, offering a preclinical foundation for developing treatments that could minimize the kidney damage associated with aminoglycoside antibiotics. By targeting multiple mechanisms of kidney injury, Parthenolide phytosomes could have broader implications for treating oxidative stress-related renal diseases.
Reference:
Albalawi, Rawan S., et al. “Parthenolide phytosomes attenuated gentamicin-induced nephrotoxicity in rats via activation of Sirt-1, Nrf2, OH-1, and NQO1 axis.” Molecules 28.6 (2023): 2741.